- The duplicated chromosomes are segregated and distributed into two daughter nuclei.
- The M phase has several distinct phases:
- Prophase
The chromatid coils and supercoils, making it more compact, the nuclear envelop dissolves.
This begins when the chromosomes have reached their maximal contracture. They move to the equatorial plate of the cell and the spindle forms.
This is triggered when a large protease called separase becomes activated following destruction of its inhibitory regulator. Each centromere divides and the new chromosomes begin to move towards opposite poles of the spindle.
The chromosomes reach the poles of their respective spindles, the nuclear envelope reforms, chromosomes uncoil into chromatin form, and the nucleolus reforms.
This is the process where one cell splits off from its sister cell. Whereas mitosis is the division of the nucleus, cytokinesis is the splitting of the cytoplasm and allocation of the golgi, plastids and cytoplasm into each new cell.
- Interphase is the stage in the life cycle of a cell where the cell grows and DNA is replicated.
- Each chromosome has its own pattern of DNA synthesis, and some segments replicate early (e.g. hours).
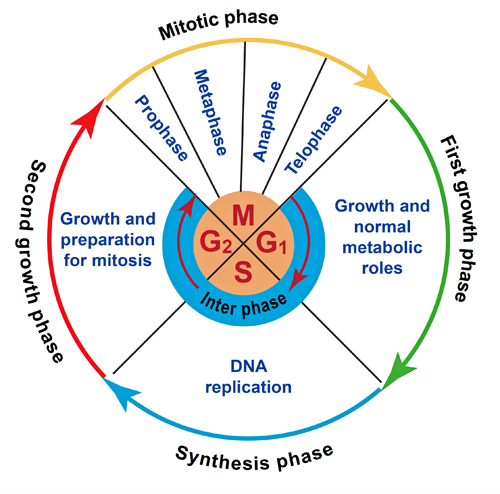
Figure 1. The cell cycle