- Wear particles are phagocytosed by macrophages releasing soluble pro-inflammatory mediators (cytokines, prostaglandins).
- Particles 0.1–10 μm are biologically active.
- Mediators released near to bone cause osteolysis by stimulating osteoclasts to release oxide radicals and hydrogen peroxide, which resorbs bone.
- Results in aseptic loosening and failure.
- Osteolysis is self-sustaining. As it loosens abrasion and fretting occur which increases the debris and wear particles.
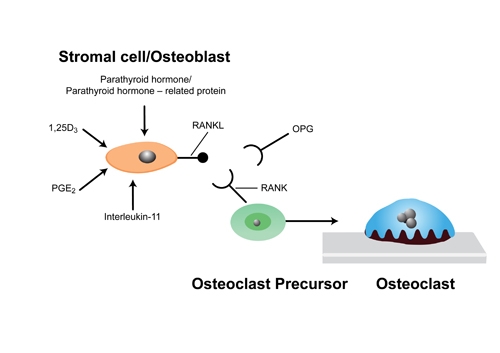
Figure 4. Cellular basis of Osteolysis
Risk factors for osteolysis
- These can be broadly divided into patient, surgical and prosthesis-related factors.
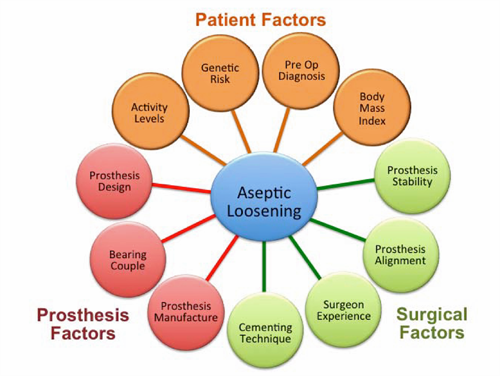
Figure 5. Summary of risk factors that influence the development of aseptic loosening
Classification of loosening
- The progressive development of radiolucent lines suggest loosening.
- This needs to be differentiated from age-related expansion of the femoral canal and cortical thinning (no sclerotic line).
- Loosening is also more irregular, with variable areas of cortical thinning and ectasia.
Classic reference1
- DeLee JG, Charnley J. Radiological demarcation of cemented sockets in total hip replacement. lin Orthop. 1976; 121: 20–32.
- DeLee and Charnley described a simple radiographic zonal classification system for the assessment of acetabular osteolysis.
- Acetabular cup loosening was assessed around the circumference of the socket and categorised into three zones (types I, II, or III) in the anteroposterior (AP) film.
- Loosening was diagnosed when a change in the position of the component had occurred or when a continuous radiolucent line wider than 2 mm was noted.
- Loosening of a cemented acetabular component is usually assessed using the criteria of Hodgkinson et al.2The DeLee and Charnley classification provides for a descriptive analysis of the zone in which the osteolysis is present.
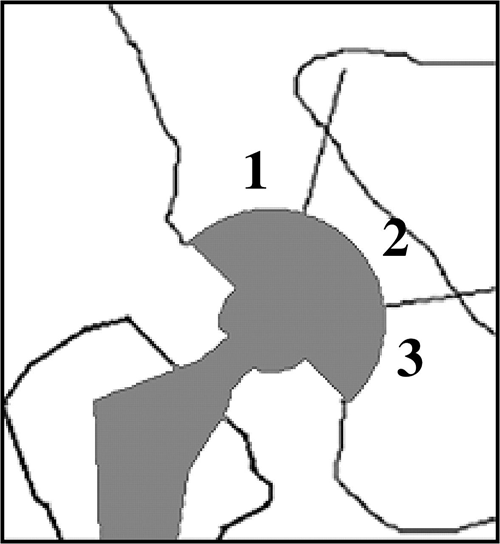
Figure 6. DeLee and Charnley Zones
Classic reference3
- Gruen TA, McNeice GM, Amstutz HC. “Modes of failure” of cemented stem-type femoral components: a radiographic analysis of loosening. Clin Orthop 1979; 141: 17–27.
- Previous attempts made to standardise the assessment of radiographs so that rates of radiological loosening at different centers could be compared had been incidental and not performed witha large series of patients.
- Gruen et al. developed a widely used system in which the femoral component interface is considered in seven zones. These allow the location of cement fractures and of lucent lines either at the cement–bone or the cement–prosthesis interface. It is the progressive changes that are seen in serial radiographs that are important in diagnosing femoral stem loosening.
- Gruen and colleagues also comprehensively review the four Gruen mechanical modes of cemented femoral stem failure.
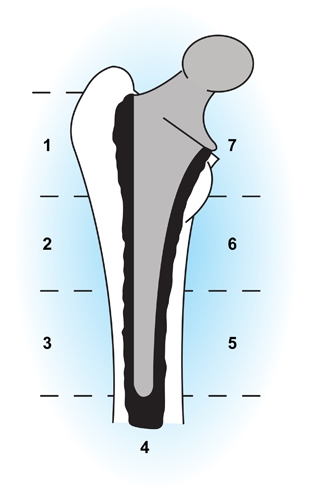
Figure 7. Gruen radiographic classification of femoral stem loosening
- The four modes of femoral stem failure are:
- Mode Ia is stem pistoning within the cement, which occurs secondary to an incomplete cement mantle or loss of proximal medial cement support.
- Mode Ib consists of stem/cement subsidence within bone. This mode of failure is most familiar to orthopedic surgeons evaluating radiographs of loose total hip replacements.
- Mode II is medial midstem pivot, which is characterised by medial migration of the proximal stem coupled with lateral migration of the distal stem tip. It is caused by weak proximal/medial (calcar) support and lack of distal cement support.
- Mode III failure consists of medial lateral toggle of the distal stem due to lack of distal stem support. This is the windshield type of loosening.
- Mode IV failure is cantilever fatigue failure characterised by partial or complete loss of proximal support with subsequent medial migration of the proximal stem while the distal end remains rigidly fixed in cement. This mode of failure can be recognised early by radiolucencies developing along the proximal lateral cortex (convex) surface of the stem.
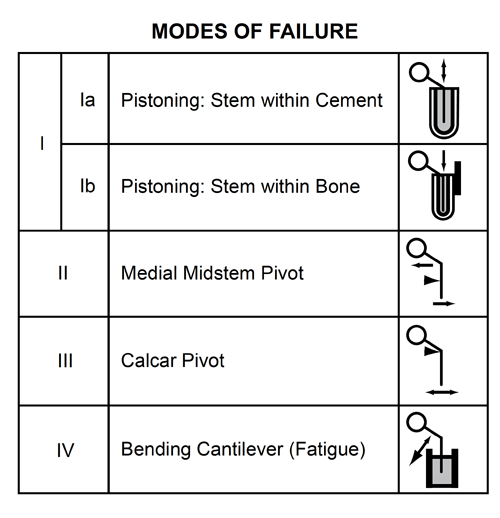
Figure 8. Modes of cemented femoral stem failure according to Gruen
Classic reference4
- Harris WH, McCarthy JC, O’Neill DA. Femoral component loosening using contemporary techniques of femoral cement fixation. J Bone Joint SurgAm 1982; 64(7): 1063-1067.
- Harris et al. proposed a radiological classification system for femoral stem loosening. Three categories were defined: definite loosening, probable loosening and possible loosening.
- Definite loosening:
- Subsidence of the component.
- Fracture of the stem.
- Cement mantle fracture.
- Radiolucent line between the stem and cement mantle not present on the immediate postoperative radiograph.
- Probable loosening:
- Radiolucent line at the bone/cement interface that is either continuous or over 2 mm wide at some point.
- Possible loosening:
- Radiolucent line at the cement/bone interface between 50 and 100% of the total bone/cement interface not present on the immediate postoperative radiograph.
- Harris later abandoned the radiographic criteria for probable and possible loosening. Studies of well-functioning, long-term mechanically stable femoral components retrieved at post mortem had shown that most cement–bone radiolucencies represent endosteal remodelling rather than disruption of implant fixation. Harris felt that the radiographic findings leading to the diagnosis of probable or possible loosening were no longer valid or useful.
Other modes of implant failure
- Instability
- Infection
- Periprosthetic fracture